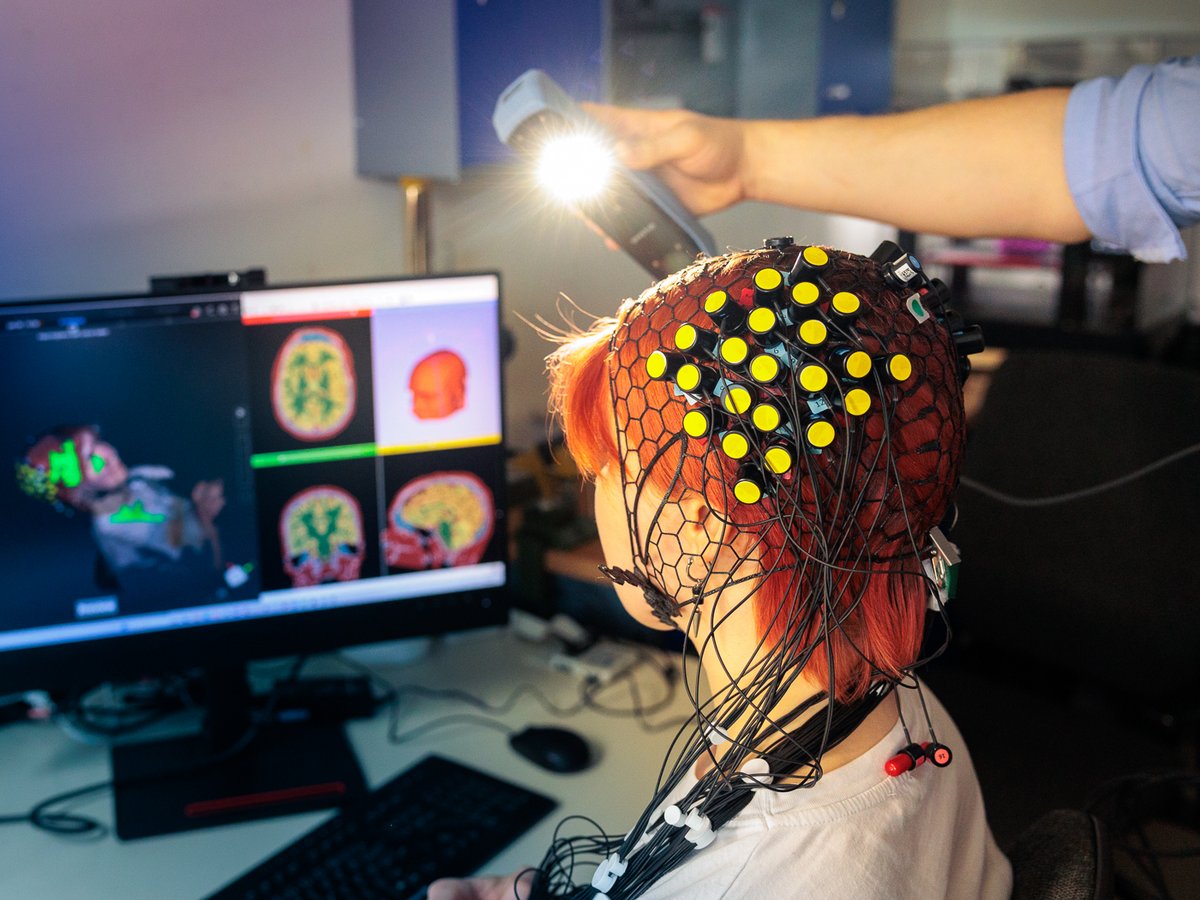
EXPEDITION NEUROTECHNOLOGY
A BIFOLD Research Team Explores AI-Driven Brain Research for Everyday Life
In 1816, the Parisian physician René Laënnec examined a young woman exhibiting symptoms of heart disease. Typically, a doctor would place his ear directly on the patient's chest to diagnose the condition. However, given that the patient was a woman, Laënnec improvised using a paper tube. He placed one end on her chest and the other against his ear. To his surprise, he could hear the heartbeat much more clearly. This marked the foundation of what would later be known as the stethoscope.
More than 200 years later, the challenge of examining the human interior without damaging the exterior remains at the forefront of research at the Intelligent Biomedical Sensing Lab (IBS) at BIFOLD. However, the brain's activities take center stage instead of focusing on the heart and lungs. The IBS team is engaged in fundamental research to understand how the brain functions in everyday life. They are developing miniaturized, portable neurotechnology that is both cost-effective and capable of providing a personalized and holistic understanding of an individual's physical and mental health.
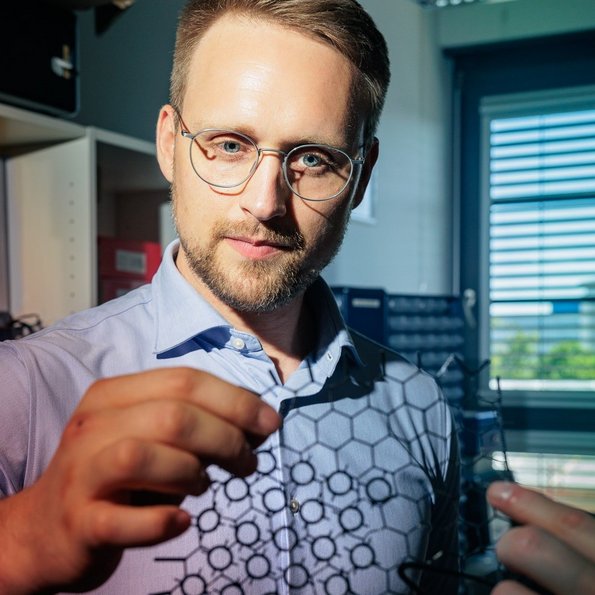
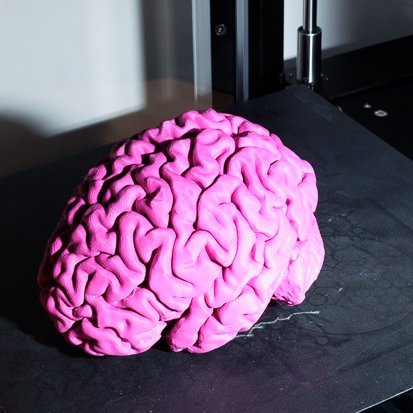
The head of the IBS Lab is Dr. Alexander von Lühmann, an expert in electrical engineering and machine learning, who earned his PhD with honors from TU Berlin in 2018. After his doctorate, von Lühmann went on to work as a scientist in Boston and later transitioned to the industry as the head of the research and development department at a medium-sized medical technology company. In 2022, BIFOLD Co-Director Klaus-Robert Müller brought him back to Berlin. "I couldn't say no to the opportunity to start my own lab here," says von Lühmann.
Three bright pink brains greet visitors as they enter the IBS Lab. The miniature models sit atop a shelf, packed in mason jars, each labeled with the names Nils, Christian, and Alex. “These are our brains,” explains Alexander von Lühmann, who goes by Alex here. “Since every brain looks different, we first have to individualize the measurement technology and determine over which brain regions our sensors are positioned for a particular test subject. To do this, we use 3D handheld scanners to create virtual models of the head. The brain models come from medical MRI scans - and, much to everyone's delight, we've also put these through a 3D printer. The results are now sitting in those jars.”
Pioneering Non-Invasive Brain Imaging
Since the early 1990s, functional near-infrared spectroscopy (fNIRS) has been used as an imaging method to measure brain activity without damaging the subject's head. When the brain is active, it consumes oxygen. To observe changes in oxygen levels, specialized (near-) infrared light (around 700–900 nm) is directed into the brain. The light penetrates a few centimeters into the head without being heavily absorbed. On its journey through the tissue, the light encounters hemoglobin, the red blood pigment that binds and transports oxygen. Hemoglobin absorbs near-infrared light, and the absorption varies depending on how much oxygen it carries. When a brain region is active, it requires a large amount of oxygen-rich hemoglobin, supplied through a process known as "neurovascular coupling." These changes in concentration can be measured by the specific optical detectors in an fNIRS cap. From the measurement results, conclusions about the underlying brain activity can be drawn. The fNIRS cap is, metaphorically, what Laennec’s paper tube was for listening to heart sounds.
3D brain images are used to precisely position the cap's measuring instruments, known as optodes (like electrodes, only optical). For individual placement, a flexible cap is needed, like the so-called "ninjaCap", which von Lühmann and others developed. This is a type of net cap made from black, flexible plastic that can be produced using a 3D printer. Observing brain activity under everyday conditions presents many new challenges. The measured signals are influenced by factors such as heartbeat, breathing, or movement, as well as environmental factors. Problem: How do you identify "real" signals from the brain when many processes are happening simultaneously and overlap the signals of interest? "Our approach is based on combining various methods – such as neurological techniques like functional near-infrared spectroscopy (fNIRS) or electroencephalography (EEG), complemented by sensors for physiology in the body and physical methods using cameras or motion sensors. And we use machine learning to decompose the signals, to improve their quality, and analyze them," explains Alex.
From Circuit Boards to Brain Caps
The IBS lab radiates maker space charm in just a few square meters. On the left, there are floor-to-ceiling cabinets packed with electronic components, measuring devices, and a repurposed pizza oven used to "bake" electronic circuit boards. On the right is a cluster of workstations: a desk, a workbench, and the 3D printers. At a precision soldering station sits Christian Tesch, bent over a prototype of a wearable head cap called M3BA that simultaneously measures brain activity using fNIRS and EEG. "One of my tasks is to ensure that the different tools we use and partly develop ourselves work together seamlessly," Christian explains.
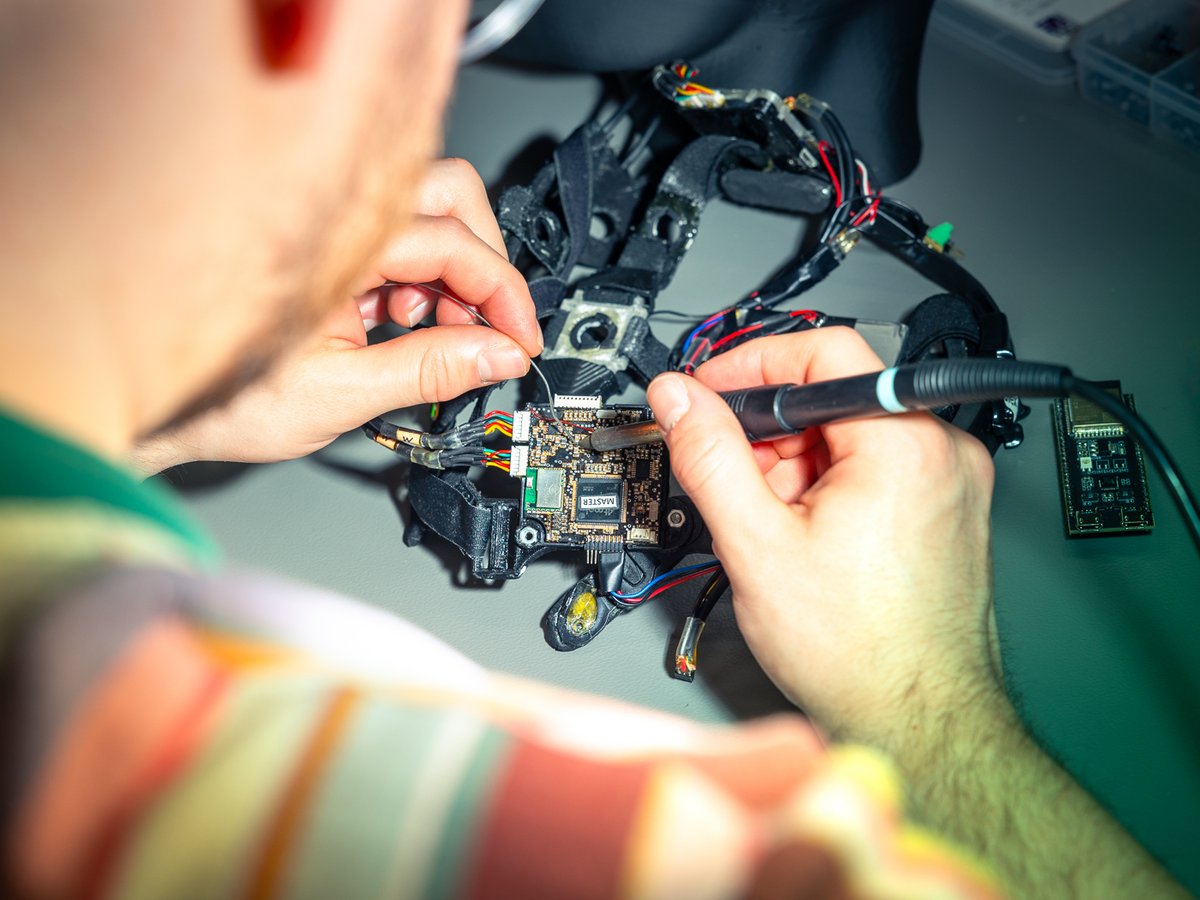
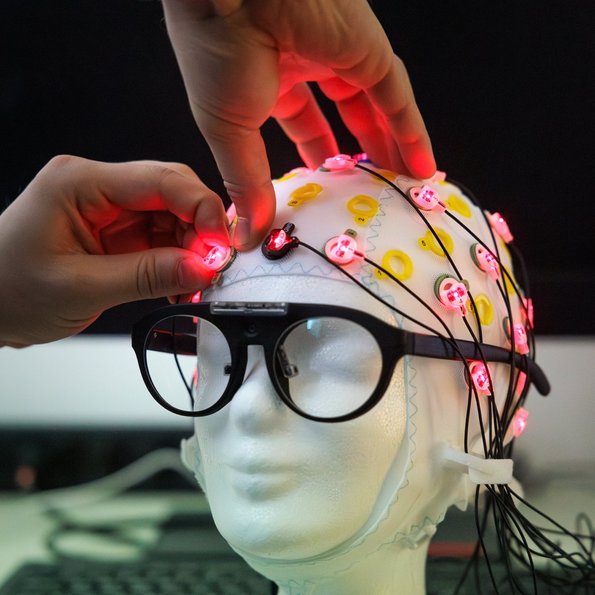
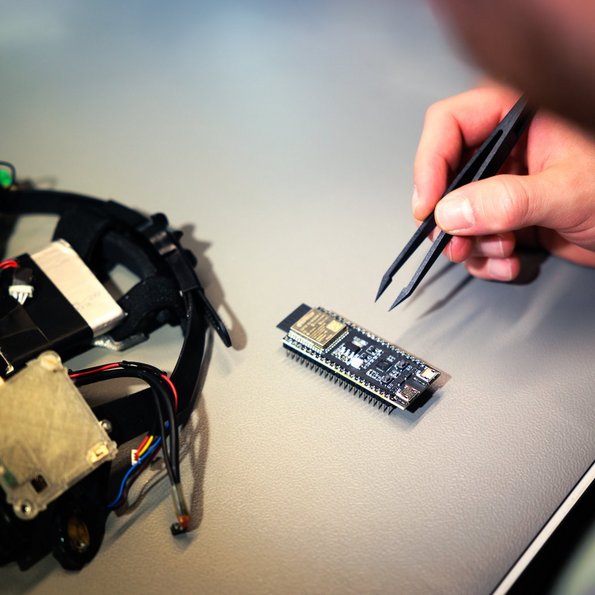
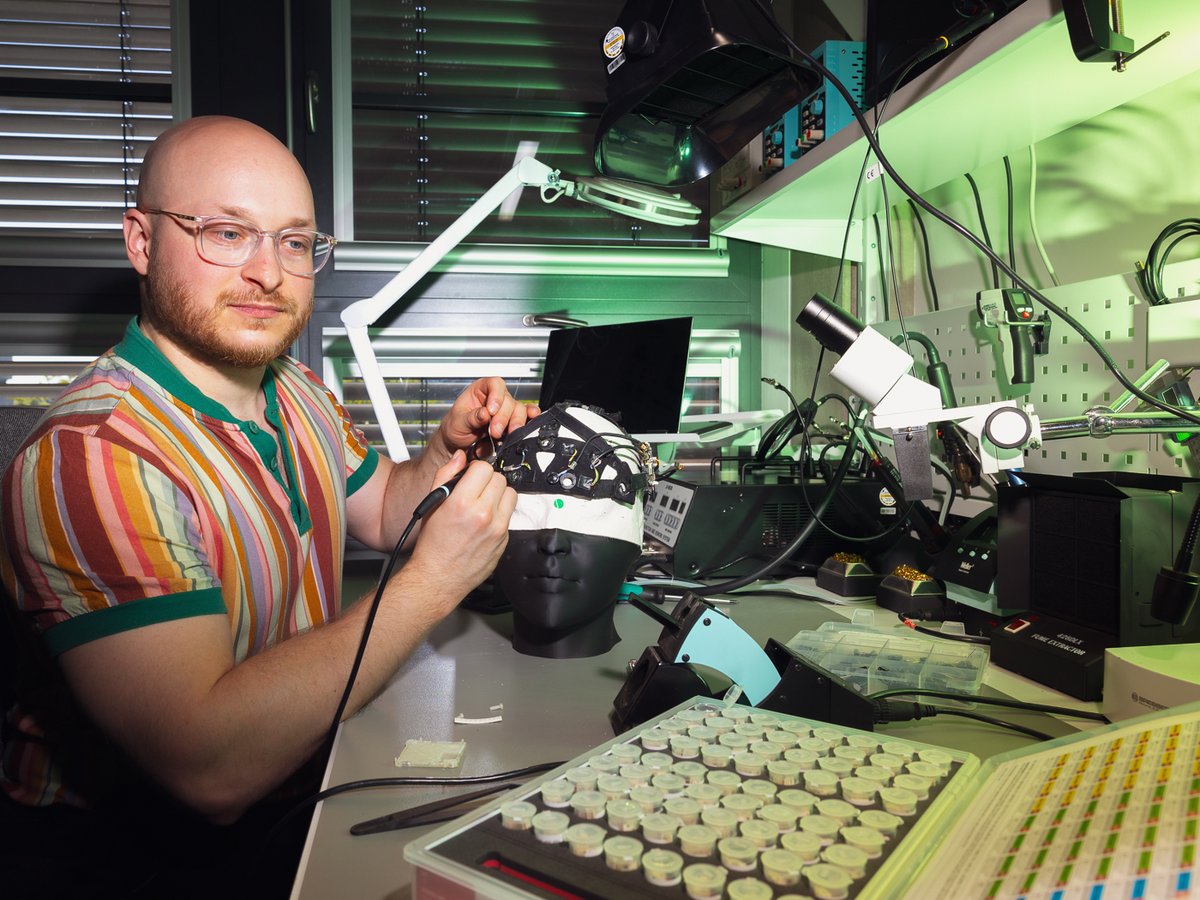
Next to Christian is a desk covered in a tangle of cables. Sitting there is Masha Iudina, a Computational Neuroscience student at TU Berlin and part of the IBS team until June 2024. She’s holding a Styrofoam head, fitting it with a ninjaCap. On the net, she attaches various small, black cylinders with yellow lids, each only a few centimeters in size. Some of these cylinders serve as light sources for near-infrared light, while others are detectors or photodiodes capable of receiving this light. Light sources and photodiodes are called Optodes. The network connecting the Optodes is called the Optode Bundle, which in turn is connected to the so-called fNIRS Imager. This modest white box converts the analog signals from the light sources and detectors into digital data and records it. The information is then transferred to the computer, where the data is stored and analyzed. Weighing just around two kilograms, the Imager is light enough for mobile applications and runs for six hours on battery power.
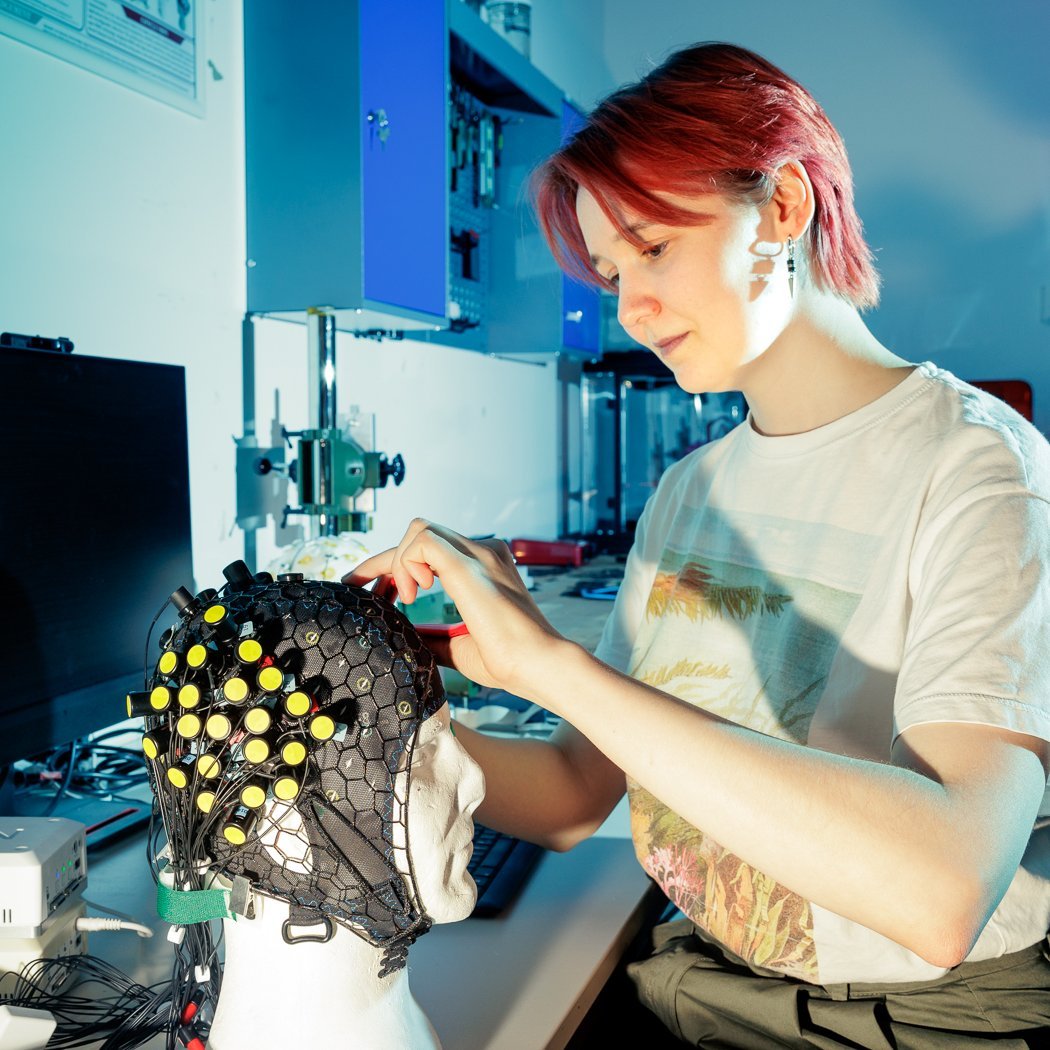
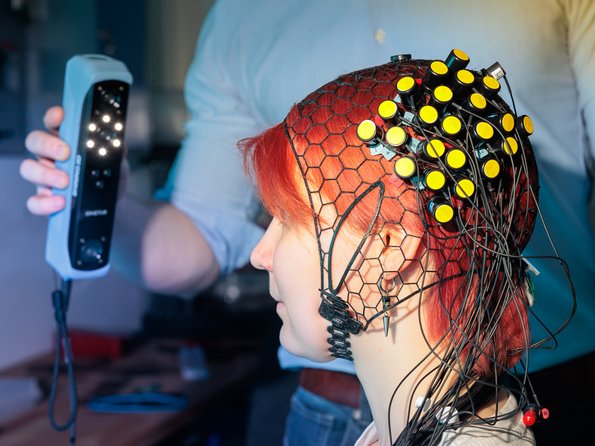
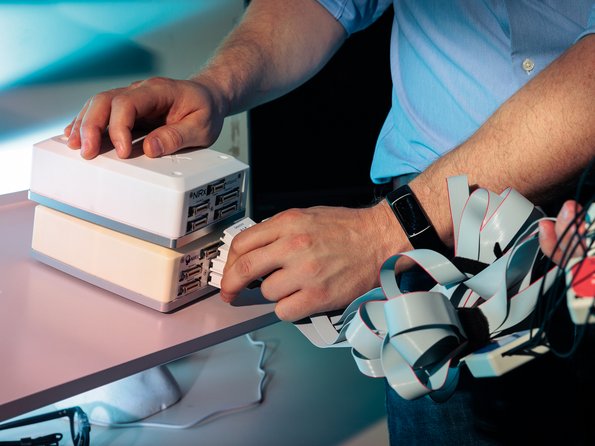
Hardware-Machine Learning Co-design
The unique selling point of the IBS Lab lies in its hardware-machine learning co-design. In addition to developing the appropriate measuring devices, the lab also creates the corresponding methods and a specialized software toolbox. In the long term, this software toolbox aims to integrate various measurement techniques—such as fNIRS and Diffuse Optical Tomography (DOT), Electroencephalography (EEG), Oximetry, and Electromyography, Electrooculography, and Electrocardiography (ExG). Additionally, it aims to simplify the connection of these measurement data with existing and new machine learning models. The specialized algorithms developed by the IBS team are designed, for example, to predict how measured brain signals are related to mental tasks or physical movements. Other algorithms work on identifying patterns within the complex mixtures of signals that could provide new insights into brain-body interaction. Most of these algorithms are still in their early stages and require further development - and BIFOLD offers the perfect environment for this advancement.
"Since April 2023, we have been working on an open-source software toolbox based on Python called CEDALION," explains Dr. Eike Midell. Trained as an astroparticle physicist, he leads development of this software at the IBS Lab. During his Ph.D., he used optical methods to study elementary particles and neutrinos at the South Pole. Today, he uses similar optical techniques to explore how brain activities can be measured with precision.
"Our vision is that one day, our tools will be an integral part of everyday clinical practice - perhaps as commonplace as the stethoscope on a doctor's coat. In such a future, large and expensive MRIs will have lost their monopoly as the primary imaging technique. On the one hand, this will allow us to learn more about the healthy brain in its natural environment. On the other hand, we might be able to better assist people with neurological disorders: through continuous diagnosis and monitoring, for example, we can provide feedback on the effectiveness of treatments - whether pharmacological, physiological, or psychotherapeutic - in everyday life, giving doctors and researchers more accurate insights."
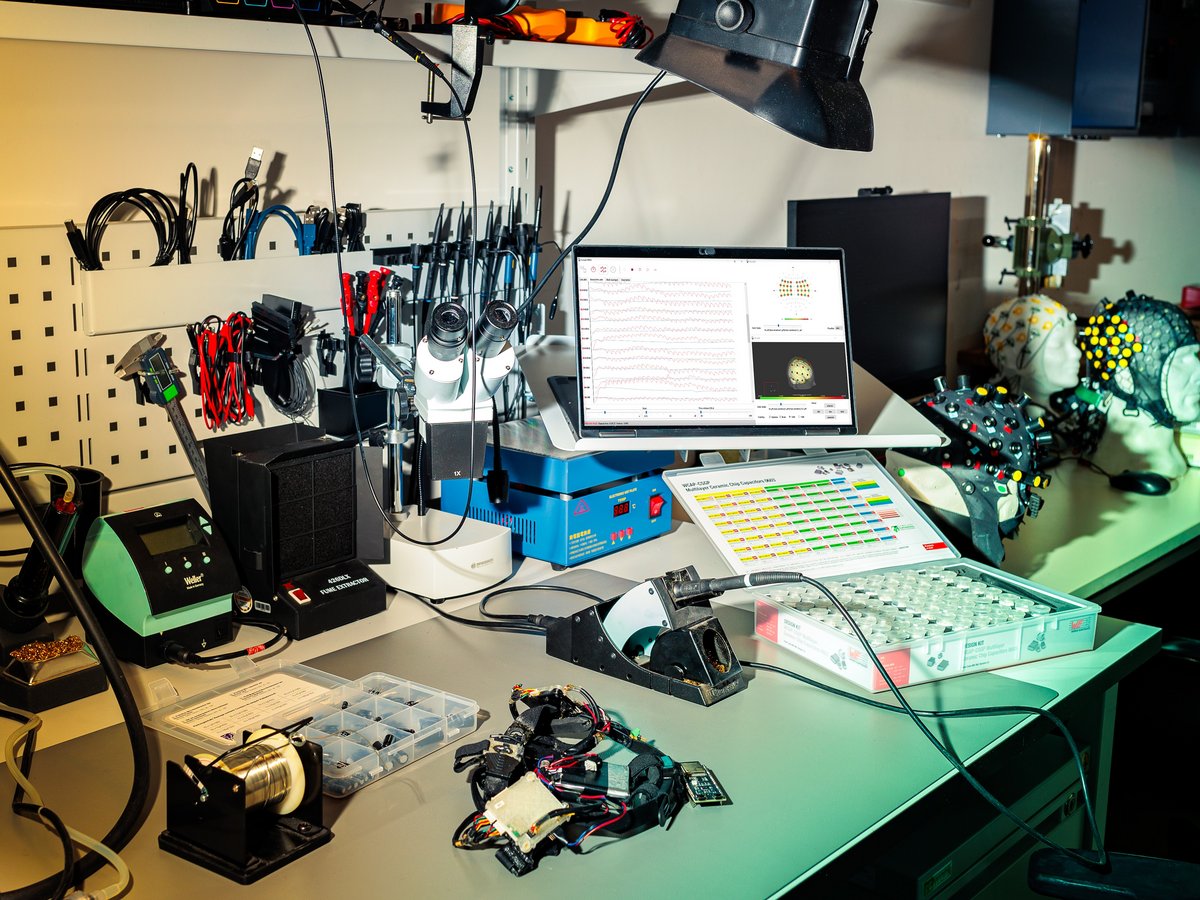